Distant galaxies are dominated by gas and stars - where is the Dark Matter?
Over the past few decades, many different studies of galaxies in the local universe have shown the existence and importance of so-called “dark matter”. While normal, or “baryonic”, matter can be seen as brightly shining stars or luminous gas and dust, dark matter interacts with normal matter only through gravity. In particular it is responsible for flat rotation curves in spiral galaxies, i.e. rotation velocities that are constant or increasing with radius.

Observations of the six galaxies with KMOS and SINFONI. For each galaxy, the left column shows the distribution of the total surface brightness in the Hα line. The right column gives the velocity map. All galaxies show a clear rotation pattern, with blue areas moving towards the observer and red parts away from the observer.
An international team of astronomers, led by Reinhard Genzel at the Max Planck Institute for Extraterrestrial Physics, have now obtained deep imaging spectroscopy of several hundred massive, star-forming galaxies in the distant universe (at redshift 0.6 to 2.6). This allowed the researchers to extract the rotation curves, which give valuable constraints on the mass distribution out to the edge of the visible disk for both baryonic and dark matter at the peak of cosmic galaxy formation 10 billion years ago. For six galaxies with the highest quality data, the scientists were able to obtain individual rotation curves, while they used a novel stacking approach for about 100 galaxies to constrain an average, representative rotation curve.
“Surprisingly, the rotation velocities are not constant, but drop with radius,” states Reinhard Genzel, first author of a Nature publication about the result. “The reason for this is twofold: First, most of these early massive galaxies are strongly dominated by normal matter with dark matter playing a much smaller role than in the local universe. Second, these early disks were much more turbulent than the spiral galaxies we see in our cosmic neighbourhood, so they did not need as much circular motion to be dynamically supported.”
Both effects seem to increase with redshift, which means that they are more important at earlier cosmic times. This suggests that in the early Universe, about 3 to 4 billion years after the Big Bang, the gas in galaxies had already very efficiently condensed at the centre of large dark matter halos. It took many billion years longer for the dark matter in the halo to condense as well, so it is not until later times that we see its dominant effect on the rotation velocities of modern disk galaxies. This explanation also agrees with the fact that high-redshift galaxies were much more gas rich and compact compared to low-redshift galaxies. Such a high gas fraction helps to dissipate angular momentum and to drive the gas inwards.
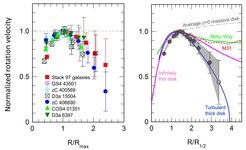
The plot on the left shows the normalised rotation curves of the galaxies in Fig 1 as well as the average rotation curve from a further 100 galaxies (filled red squares). All velocities drop after reaching a maximum value.
In the plot on the right, the individual data points have been binned to facilitate comparison to local rotation curves (our Milky Way in green, the Andromeda galaxy M31 in red). Also plotted are the theoretically expected rotation curves for a thin disk without dark matter in the inner regions and for a thick disk. It is obvious that one needs both a dark matter distribution, which is not concentrated in the centre of the galaxy, and the turbulent motion in a thick disk to explain the observations.
“We have to be very careful when comparing these early massive and gas-rich rotating galaxies to the ones in our local universe,” cautions Natascha Förster Schreiber, co-author for all four studies. “Present-day spirals, such as our Milky Way, require additional dark matter in various amounts. On the other hand, local passive galaxies – which are dominated by a spheroidal component and are the likely descendants of the galaxies in our study - show similarly low dark matter fractions on galactic scales.”
Two additional studies of 240 star forming disks support these findings. Detailed dynamical modelling shows that, while baryons account for typically 56% of the total mass fraction in all galaxies, they completely dominate the inner matter distribution for galaxies at the highest redshifts. The other analysis used the same data in the framework of the Tully-Fisher relation describing a tight relationship between the rotation velocity of a galaxy and its mass or luminosity. Again, the observations show that massive star-forming galaxies at high redshift have a higher baryon fraction out to the outer disk than at lower redshift.
“You can do the arithmetic,” explains Stijn Wuyts from the University of Bath, a co-author on all four papers, “the dynamics reveal the total mass present. Subtract from that what we see in the form of stars and gas, and there really is not much room left for dark matter within these early disk galaxies. The dropping rotation curves are not only in line with these results, they provide a more direct indication of the baryon dominated nature, especially to researchers that have a healthy scepticism about the accuracy with which one can measure the amount of stars and gas in these distant objects."
Note:
The data analysed were obtained with the integral field spectrometers KMOS and SINFONI at ESO’s Very Large Telescope in Chile in the framework of the KMOS3D and SINS/zC-SINF surveys. It is the first time that such a comprehensive study of the dynamics of a large number of galaxies spanning the redshift interval from z~0.6 to 2.6, or 5 billion years of cosmic time, has been carried out. The team analysing and interpreting the data in the Nature publication consists of R. Genzel, N.M. Förster Schreiber, H. Übler, P. Lang, L.J. Tacconi, E. Wisnioski, S. Belli, A. Burkert, J. Chan, R. Davies, M. Fossati, A. Galametz, O. Gerhard, D. Lutz, J.T. Mendel, E.J. Nelson, R. Saglia and K. Tadaki at the Max Planck Institute for Extraterrestrial Physics (MPE), T.Naab at the Max Planck Institute for Astrophysics (MPA), R. Bender, A. Beifiori and D.Wilman at the University Observatory Munich, S. Wuyts at the University of Bath, T. Alexander at the Weizmann Institute of Science, G. Brammer at the Space Telescope Science Institute, C.M. Carollo and S. Tacchella at the ETH Zürich, S. Genel at the Center for Computational Astrophysics, I. Momcheva at Yale University, A. Renzini at the Astronomical Observatory of Padua, A. Sternberg at Tel Aviv University.
KMOS was developed and built by a balanced consortium of UK (University of Durham, University of Oxford, UK Astronomy Technology Centre Edinburgh (ATC)) and German institutes (University Observatory Munich, Max Planck Institute for Extraterrestrial Physics) in partnership with the European Southern Observatory. Principal Investigators are Ray Sharples (University of Durham) and Ralf Bender (University Observatory Munich/Max Planck Institute for Extraterrestrial Physics).